Research Areas
The Gagliardi Group develops novel quantum chemical methods and applies them to study phenomena related to sustainable energies, with special focus on catalysis, photochemistry, spectroscopy, molecular qubits and magnets, actinides, metal organic frameworks and covalent organic frameworks. We are interested in modeling molecular species, materials, and interfaces. We develop electronic structure theories, machine learning protocols, and combine quantum and classical simulation techniques.
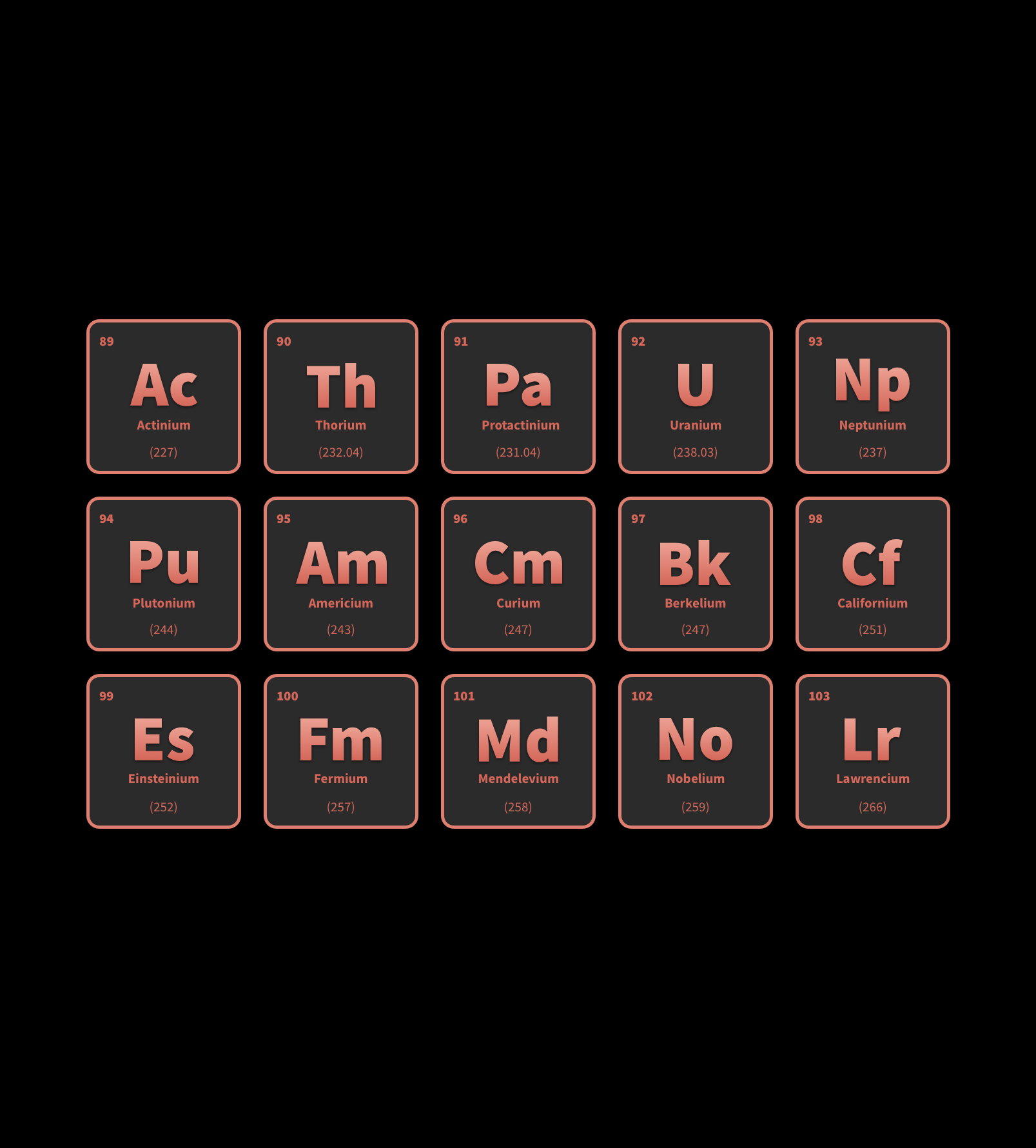
Actinides
We model actinide and transactinide chemistry, with the aim of understanding the electronic structure and chemical bonding of molecular species both in their ground and excited states, as well as the processes that govern the formation of actinide-based materials relevant to the spent nuclear fuel reprocessing and to nuclear security. In our technique, we utilize electronic structure methods, classical simulation techniques and data science. Read more
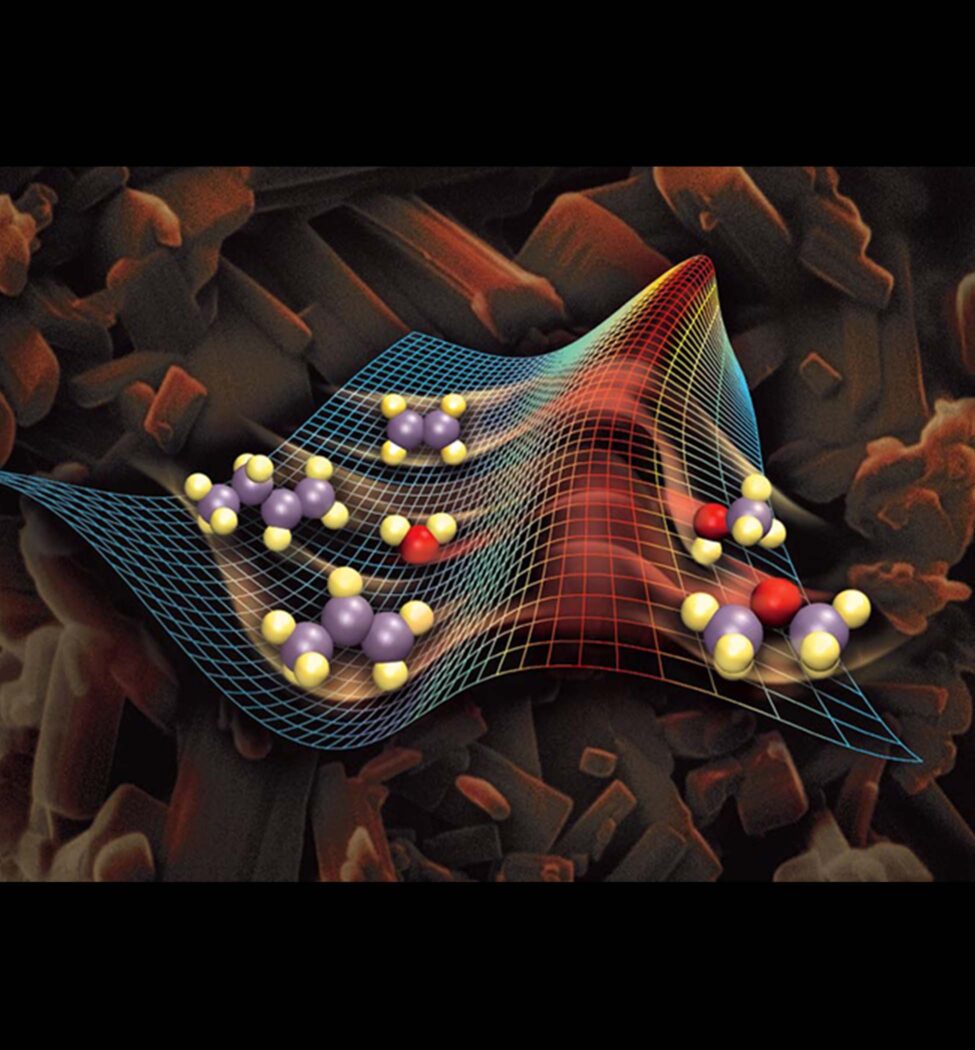
Catalysis
We model catalysis, spectroscopy and photochemistry of molecular systems containing transition metals and catalytic phenomena involving metal or metal-oxide clusters attached to a support, such as metal-organic frameworks (MOFs). Examples of recent published studies have examined C-H bond activation in methane, electrocatalytic hydrogen evolution, and methane oxidation to methanol. We utilize density functional theory and wave function-based methods to study synthesis and catalytic reactions that aid in the characterization of the materials, combined quantum mechanical/molecular mechanics methods and data science. Read more
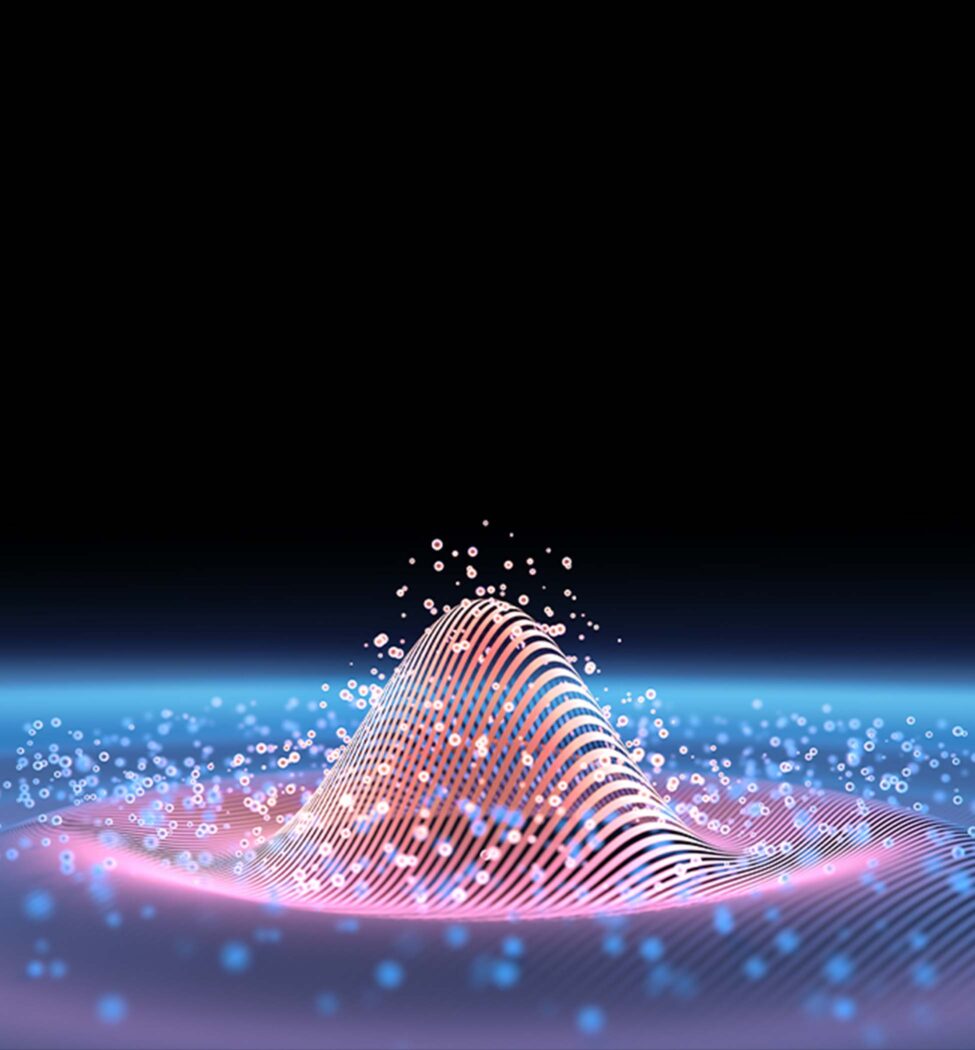
Classical Simulations
Classical force field-based simulation methods overcome the system size limitations of quantum chemical simulation methods to model several phenomenal, for example, gas adsorption and storage phenomena in porous materials, and, more generally, the statistical behavior of systems in a condensed phase. Our group develops force fields derived from first-principles data to accurately describe the governing host-guest interactions. Classical simulations using these ab initio-derived force fields are employed to investigate the adsorption and diffusion behavior of gases and industrial solvents in the adsorbent for various applications including carbon capture, hydrogen separation, and water harvesting and actinide chemistry in solution. Read more
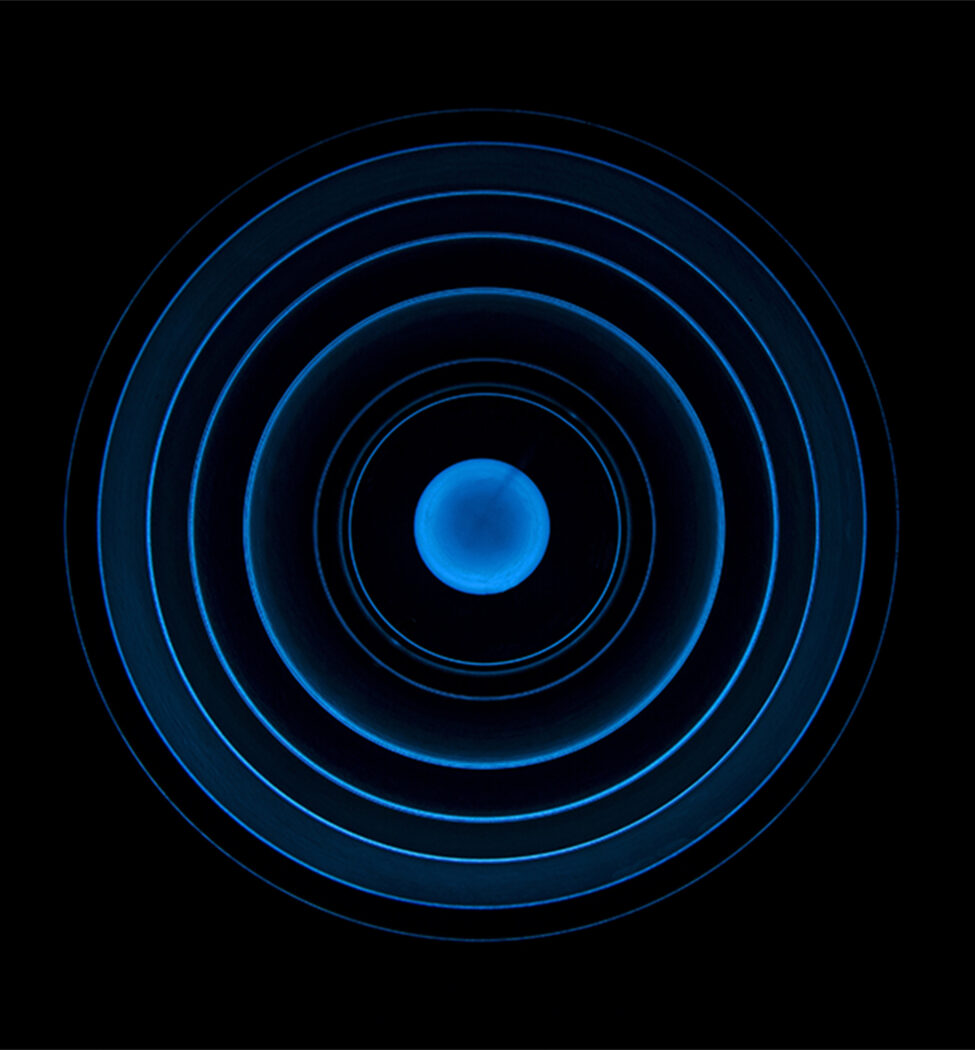
Electronic Structure
We develop electronic structure methods to model strongly correlated systems, including organic compounds, transition-metal, actinide and lanthanide complexes, and qubit candidates. We advance embedding methods that allow us to simulate very large molecules and condensed-phase systems and formulate multireference density functional theories to model them. Read more
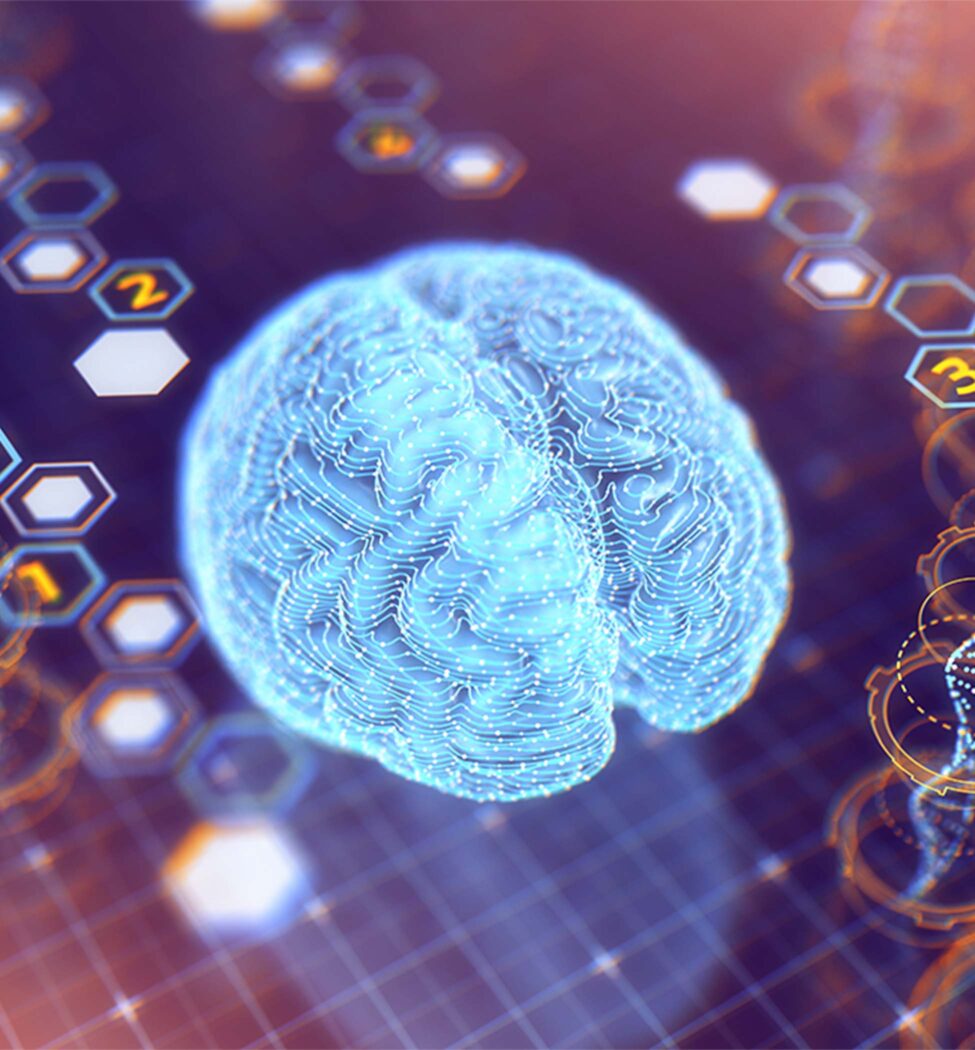
Machine Learning
We use machine learning algorithms to parameterize models to make useful predictions from theoretical and experimental data. Examples include predicting new experimental conditions for high-yield catalysis and developing parameterized approximations for electronic structure methods. Chemistry has a long and rich history of using parameterization from data to understand the world around us and machine learning is the modern continuation of this tradition. Read more
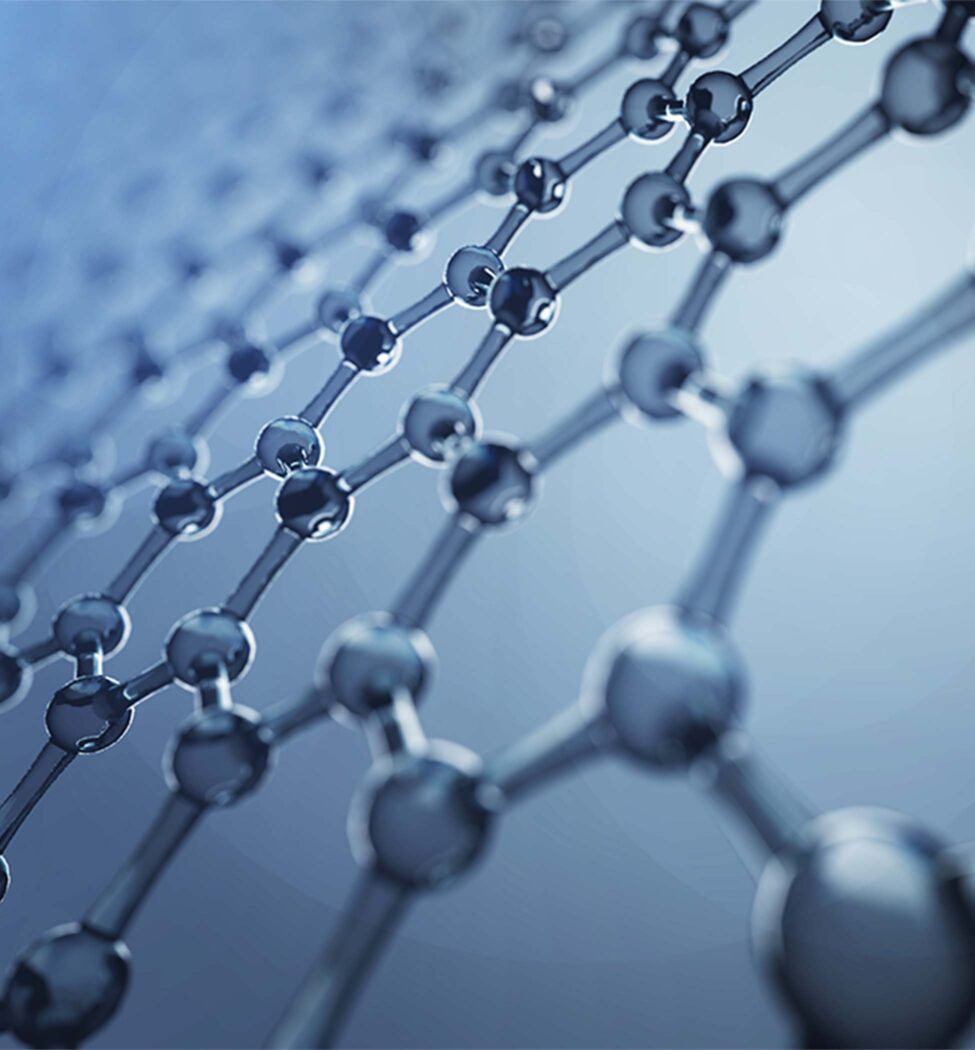
Reticular Frameworks
Metal-organic frameworks (MOFs) are crystalline, porous, extended structures, which link transition metal ions by anionic organic linkers. Due to their modular and highly tunable nature, MOFs have received a significant amount of attention from the scientific community for applications in gas separation and storage, photochemistry and catalysis. Our group collaborates with experimentalists to investigate the nature of MOFs and their effectiveness in various applications by employing both quantum and classical simulations. Within this same effort, we also explore covalent organic frameworks (COFs), which are entirely organic crystalline, porous, extended structures formed by organic building units linked by strong covalent bonds and can be employed for numerous applications. Read more
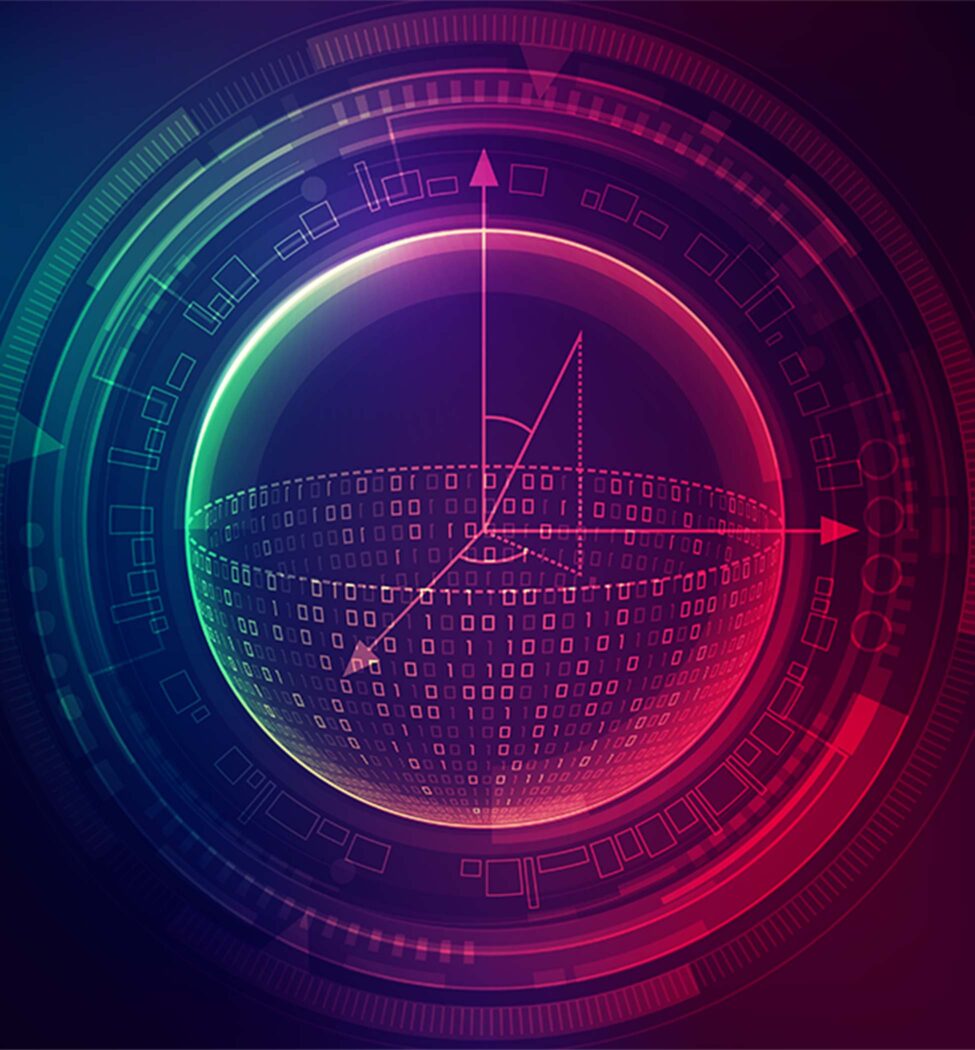
Molecular Qubits and Magnets
The design of magnetic materials is relevant to information storage and quantum computing. In partnership with experimental research groups, we develop electronic structure protocols to study magnetic properties of molecular systems and materials and to assess their potential as spin-qubits. Using state-of-the-art multiconfigurational methods, we predict zero-field splittings, relaxation barriers and magnetic susceptibility of such systems. Read more
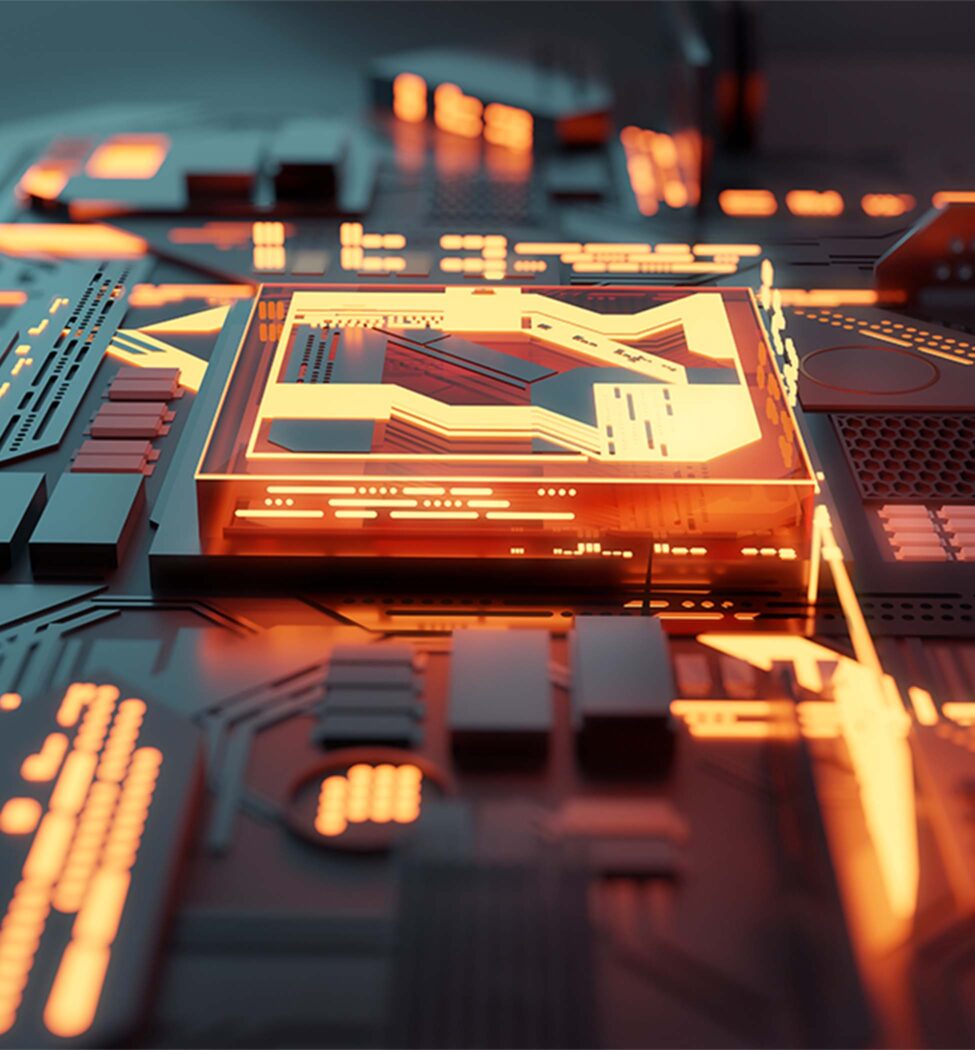
Quantum Computing
Quantum computers are inherently powerful tools in quantum chemistry because of their ability to prepare superpositions of states and perform unitary transformations. While quantum algorithms like the quantum phase estimation (QPE) offer exponential speedup over their classical equivalents, the practical limitations of the quantum hardware restrict the range of applications to simple problems. We design chemically guided quantum algorithms that combine the power of quantum computing and insights from classical electronic structure theory to make these calculations computationally efficient. Our recently published method – LAS-UCC – leverages the local nature of electron correlation to scale better than the conventional QPE algorithms. Read more
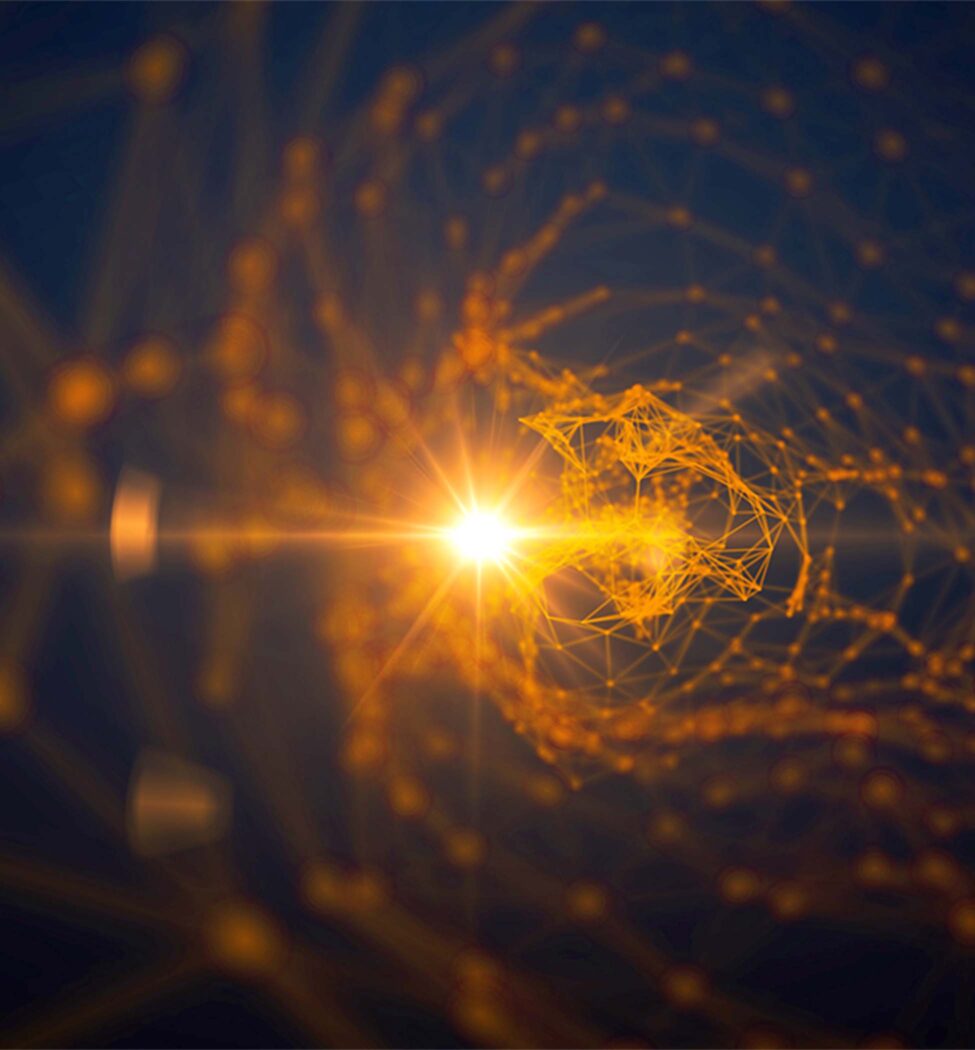
Photochemistry
Photoinduced physical and chemical processes in molecules are central to many applications in the chemical industry including photoredox catalysis, organic synthesis, and design of photosensitizers and photoluminescent materials. We use ab initio molecular dynamics simulations and statistical kinetic theories coupled with multireference electronic structure methods to understand the dynamics of electronically excited states and predict their reactivity in photochemical processes. The ability to describe the mechanistic details of nonradiative relaxation allows us to guide molecular synthesis and leverage the power of external radiation to design new compounds with target molecular properties. Read more